Access to clean water is a fundamental human need, and has been described by the United Nations as a human right ‘essential to the enjoyment of life and all other human rights’. Yet the presence of contaminants in drinking water, whether biological or chemical, is widespread, causing disease and death around the globe.
The challenge is thus to find both low-cost reliable ways of analysing toxic contaminants in the field, and develop cheap and effective remediation methods. These will be particularly beneficial to developing countries with limited resources for detection of arsenic, the most toxic of them all, in water.
Arsenic-contaminated drinking water has been identified in many countries around the world including Argentina, Australia, India, Mexico, Thailand and Bangladesh. The World Health Organisation (WHO) declared the problem in Bangladesh to be ‘the largest poisoning of a population in history’, with up to 104 million people affected.
The World Health Organisation declared the problem in Bangladesh to be ‘the largest poisoning of a population in history’
Arsenic in drinking water is dangerous even at very low concentrations through cumulative exposure over a number of years. Concentrations as low as 10 micrograms per litre (or parts per billion – ppb) can be toxic in humans, causing cancer and other serious illnesses. A recent reduction in the guidelines for maximum arsenic concentration from 50 to 10 ppb by the WHO in 2006, also adopted by the US Environmental Protection Agency, has increased pressure to develop detection and remediation technology.
Reliable determination of arsenic concentrations in the lower ppb range (0-20 ppb) is difficult and is usually carried out using sophisticated and expensive lab-based techniques. Such analysis is too costly for many countries, and hence they have not been able to implement the new rules. The challenge now is to find both low-cost and reliable ways of analysing arsenic in the field in addition to finding cheap and effective remediation methods.
Chronic Exposure
The toxic nature of arsenic is notorious, with its use as a poison throughout human history well documented. However, long-term exposure to arsenic from environmental sources (chronic exposure) causes symptoms that are very different to those resulting from acute poisoning, and can result in a range of very serious health problems.
Concentrations as low as 10 ppb are responsible for causing a variety of cancers, cardiovascular and lung diseases, gastrointestinal illness and neurological disorders. Dermatological effects are also very common, with more than 1,000 people known to have developed skin disorders caused by intake of arsenic released during mining in the Ron Phibun region of Thailand alone. Such dermatological effects, due to their visibility, are often used as an indicator that chronic exposure is occurring in a given environment. Long-term exposure to arsenic has also been implicated in the impairment of children’s neurological development.
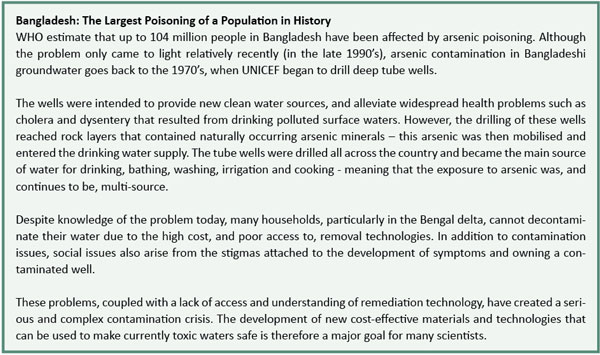
Arsenic occurs naturally in two forms, namely arsenite and arsenate. Arsenate usually exists as a negative ion (except in very acidic waters) which is chemically very similar to phosphate (ubiquitous in biology). This means that arsenate can replace phosphate in biological systems, thereby interrupting the delicate biochemistry of living cells. One such case where this can occur is ATP (adenosine triphosphate), which has an extremely important role in the storage and transfer of energy within cells. Arsenite is capable of interrupting important biochemical pathways in a different way, by binding to enzymes and disrupting their function.
The carcinogenic properties of arsenic arise from its propensity to damage DNA. The risk of developing cancer from exposure to drinking water containing 50 ppb arsenic is 100 times greater than the risk posed by any other contaminant at this level. In 2006 the WHO reduced their guidelines for safe arsenic levels from 50 to 10 ppb. Without swift action, it can be expected that future disease rates of those exposed will rise as long-term exposure to these dangerous levels continues.
The risk of developing cancer from exposure to drinking water containing 50 ppb arsenic is 100 times greater than the risk posed by any other contaminant at this level
Adsorbing Arsenic
Naturally occurring arsenic is generally found either adsorbed to the surface of clays and minerals, or is actually incorporated within the mineral structure. It can be mobilised into groundwater through the dissolution of the supporting mineral or through desorption from the surface.
The two naturally occurring forms of arsenic – arsenate and arsenite – possess quite different physical properties. The type of the water source in question determines which form is found: in oxygen-rich waters arsenate (+5 oxidation state – AsV) prevails, and in oxygen-poor waters arsenite (+3 oxidation state – AsIII) dominates. In most natural waters, the negatively charged arsenate can stick to positively charged surfaces such as iron oxide minerals (adsorption), whilst neutral arsenite is much less readily adsorbed by surfaces.
Thus, the general idea of remediation technology is that materials such as iron oxide are used as adsorbents to remove arsenic from the water. For example, the large purification tanks at the Severn Trent Water arsenic adsorption plant, Worcestershire, contain an iron oxide material. As contaminated water is passed through the adsorbent, the arsenate sticks to the surface and is removed from the water. However this method remains more effective for removing arsenate than arsenite. Pre-treatment of water, either to convert arsenite to arsenate or to lower the pH (which both improve uptake of arsenic) is common.
Iron oxides have also been exploited in much cruder filters, designed for use in the developing world and particularly rural areas, where the remediation techniques (including point-of-use, community and aquifer wide removal plants) are much more rudimentary. Examples of these include household systems such as the SONO filter, developed in 2001, which consists of stacked plastic buckets filled with an iron matrix, coarse sand, charcoal, brick chips, and fine sand. Up to 20 litres of water can be filtered per hour using this filter whose lifetime can sometimes reach five years. Community removal plants, on the other hand, consist of up to 3 filters directly fitted to pump wells. However the effectiveness of both SONO filters and community devices remains unclear. A 2005 study highlighted the failure of community removal plants in West Bengal to remediate arsenic levels below 50 ppb. Tests carried out for the SONO filter were more promising; results showed arsenic concentration in filtered water of <10ppb.
The general idea of remediation technology is that materials such as iron oxide are used as adsorbents to remove arsenic from the water
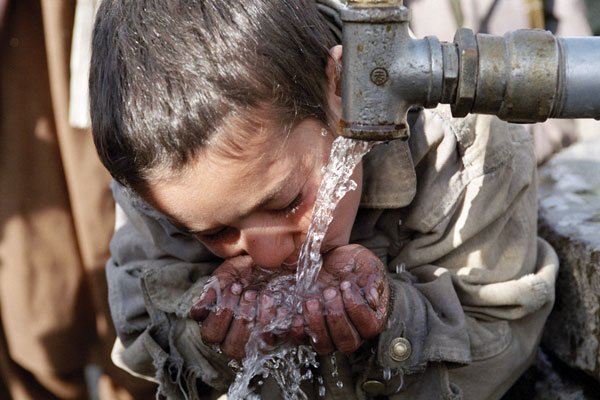
On a larger scale, subterranean arsenic removal technology (SAR) involves the pumping of aerated water back into the aquifer causing oxidation of arsenite to arsenate (as the oxygen content of the water is increased). This approach has the advantage of addressing the arsenic present in both domestic and irrigation water. However, if the planned oxidation is poorly calculated, arsenic may precipitate, leading to longer stabilisation periods in the initial stages.
Many current remediation technologies also face problems due to the presence of other chemical species in groundwater interfering with the arsenic uptake process. For example, phosphate, chemically similar to arsenate, is often found at much higher concentrations. Phosphate ions compete with arsenate at the adsorbent surface, and either cause the arsenate to desorb from the surface, or block adsorption sites, thus inhibiting arsenic removal.
Phosphate competition presents a real challenge which could be overcome either by designing new materials with a more specific arsenate binding ability or via (potentially expensive) water pre-treatment processes. However, the more complex and involved the processing and treatment stages, the less likely developing countries will be able to afford to implement the technology.
Toxic Waste
Dealing with the waste produced post-remediation raises further issues in developing countries. The disposal of arsenic remediation waste is difficult because leaching or loss of arsenic from waste can result in further contamination. Adsorbent technology often results in the formation of arsenic-containing liquid-waste that requires a high standard of waste management for proper and safe disposal. Therefore adsorbents resulting in a solid waste, that is suitable for landfill, are preferable.
Solid wastes can then be tested for their stability and leachability in various settings, and be separated into hazardous and non-hazardous substances. Wherever specific facilities are not available, this waste may be dealt with informally by spreading it on land, diluting and dispersing it into rivers or casual landfills.
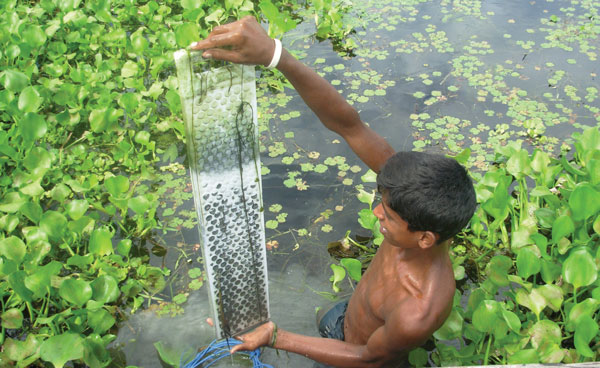
A novel method was recently proposed suggesting that arsenic remediation sludge should be mixed with cow-dung so as to form methylate arsenic which then no longer poses a risk. This is similar to the use of sludge in brick making; mixing it with clay immobilises the arsenic. Designing efficient, long lasting materials that do not require frequent regeneration (hence minimising the total volume of waste needed to be disposed of), is the best way to effectively tackle the issue of waste resulting from arsenic removal.
Clean Water for All
Research into all aspects of the arsenic problem, from detection of low concentrations to developing new remediation materials, is currently on-going at Imperial College. The aim of this research approach is to design a two-stage model in which both forms of arsenic are removed from drinking water at once.
The first stage utilises a novel titanium dioxide/iron oxide material, together with UV irradiation, to oxidise the more toxic arsenite to arsenate. This first stage serves to eliminate important competing ions such as phosphorus in order to make stage two more efficient. The second stage of remediation then focuses on binding the residual arsenate, thus bringing arsenic levels below recommended levels.
Adsorbent technology often results in the formation of arsenic-containing liquid-waste that requires a high standard of waste management for proper and safe disposal
The goal of providing clean water for all the world’s population presents an inspiring challenge, both in human and scientific terms, and is a driving force for much innovative and exciting research around the world. It is clear that arsenic contamination poses a number of important challenges to governments, regulatory agencies and researchers around the globe. Low-cost detection of low levels of arsenic is a key priority, and, while remediation technologies have been implemented with some success, implementation of efficient and affordable arsenic filters in the developing world is urgently needed. Only with combined effort from scientists, engineers, aid agencies and governments can this global problem be truly confronted.