Synthetic Biology, initiated in 2007, has quickly evolved into a fast-paced and exciting research field. Extensive future applications surrounding this diverse subject have earned it a strong reputation amongst scientists, and led to it being deemed a ‘top research priority’ by the UK government. Whilst previous centuries evolved around the harnessing of fossil fuels, and the resulting agricultural and industrial revolutions, the 21st Century has been witness to a biological revolution.
Synthetic biology combines the skills of a variety of professionals, such as mathematicians, (molecular) biologists, physicists and chemists, all collaborating in an endeavor to engineer synthetic life. This research initiative is of the greatest importance as it holds the potential to re-direct the use of fossil fuels to new platforms such as consumable and renewable energy, cure life-threatening diseases such as cancer, and even create new cell types all-together.
Like other leading fields there are a plethora of ethical questions and issues, especially surrounding some aspects of ‘creating life’. But, with so many arguments in favour of progress in this unique field, such debate largely serves to ensure safe practice for research in this area. Ultimately, the applications of synthetic biology can only be limited by our imaginations.
From the initial discovery of DNA by Franklin, Wilkins, Crick and Watson in 1953, research in this field quickly progressed, leading to Robert G. Edwards’ development of in vitro fertilization (IVF) in 1978 and the subsequent first successful conception of an IVF baby. A few decades later, in 1997, Dolly the Sheep, possibly the most famous animal in academia and beyond, was cloned by nuclear transfer heralding yet another milestone in genetic engineering.
Synthetic biology (is) described as the design and construction of new biological parts, devices, and systems, and re-design of existing, natural biological systems for useful purposes
In parallel to advances in molecular biology, the development of technological tools used for research within this field has been equally paramount to the success of breakthrough experimentation. For example, in the ‘60s, integration of chemistry and biology created the first synthetic oligonucleotide, which is short single stranded strings of DNA or RNA; this process was promptly automated by the ‘70s. The following decade gave rise to the first synthetic gene.
Over time the cost of DNA sequencing technology has plummeted almost 100,000 fold due to technological development. At the start of this century, the average cost per megabase of DNA was as high as $5,292.39 whereas now the same length can be sequenced for $0.06. Without these combined factors genetics and molecular biology could not have evolved into synthetic biology.
Synthetic biology researchers have a well-established definition for their topic, described as ‘the design and construction of new biological parts, devices, and systems, and re-design of existing, natural biological systems for useful purposes’1. To be more specific, biological parts are sections of a DNA sequence that encodes anything within the sphere of genetics, such as promoters. Promoters are a section of DNA that initiates transcription of a particular gene. The characterization of parts like promoters, contributes to the modularity of the system. By manipulating the DNA sequence of a promoter, researchers are able to produce a ‘library’ of a promoter ranging in function and strength. The ability to tailor a promoter sequence for a particular function enables very precise tuning of the gene(s) we wish to express. Scientists have since successfully reprogrammed bacteria with new machinery and abilities.
Challenges and Applications
Despite the fast-paced, novel research emerging from the field of synthetic biology, there are limiting factors that remain to be overcome. A primary example is the significant shortage of characterized key components of genetic circuits such as promoters, terminators, repressors, etc. Few have been identified, assessed and evaluated under specific conditions and parameters. Furthermore, of this minority, characterization was performed largely in prokaryotic organisms, chiefly Escherichia coli. The next step is to work with the eukaryotes, such as yeasts and mammalian cells.
An additional setback is the lack of technology that aids our ability to edit and engineer genomes, such as next generation sequencing methods. Two leading groups working on precisely this area are Isaacs and Church, from Yale and Harvard respectively. Progress on developing new technology platforms like CAGE (Conjugative Assembly Genome Engineering) and MAGE2 (Multiplex Automated Genome Engineering) is a step forward to assisting us in using high throughput and automated methodologies.
On the other hand, synthetic biology has progressed in leaps and bounds in recent years. Particular areas of focus are unsurprisingly the most pressing issues that mankind faces today, including life-threatening diseases such as cancer, tuberculosis and malaria. Other major topics of interest lie in environmental areas including non-renewable energy sources, carbon emissions and fixations. Developing new drugs from the (re)programming of prokaryotic or eukaryotic cells, and creating new, renewable energy sources, are all part of ongoing research that is well underway.
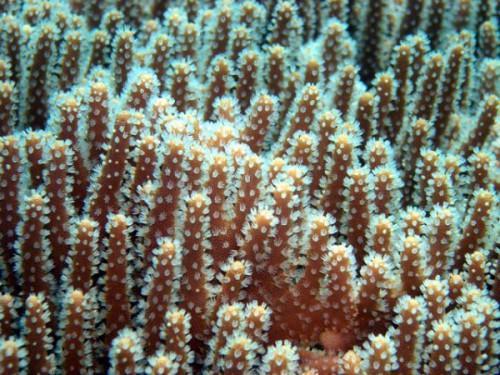
For example, Jay Keasling’s lab at UC Berkley is engineering microbial factories to counteract the effects of malaria by producing the drug artemisinin using a yeast platform to manufacture it at an affordable price, and therefore making it accessible to the people who need it most. His team is also looking to create novel and environmentally friendly compounds, as well as biofuel substitutes for gasoline, diesel and jet fuel. At Harvard University, Pam Silver is researching the rapid and predictable programming of cells of all types for a multitude of purposes. One of her research interests is to replicate the photosynthetic machinery in non-photosynthetic bacteria to engineer the fixation of carbon dioxide, and to try and exploit sunlight exposure as a stimulus for the production of useful compounds such as sugar. Her team is also working on developing memory in engineered bacteria, enabling them to report the state of the gut.
Other fascinating achievements have included the production of spider silk from goats’ milk. Randy Lewis, Professor at the University of Wyoming, has expressed the silk gene in goats in such a way that the silk is secreted with their milk. Spider silk is an exceptional material, one of the strongest known to man and more so than Kevlar and more elastic than rubber. An additional positive observation was that there was no change to the health, phenotype or quality of life of the goats.
Genome Engineering
As the understanding and knowledge of biological engineering continues to expand, so have branches of synthetic biology and other new fields. Genome Engineering is a much talked about and compelling new genre. This new niche is dedicated to creating entirely novel organisms, and as its title suggests to engineer new genomes from a bottom-up approach, as opposed to synthetic biology that takes on a top-down approach. As the newest chapter in synthetic biology, this field is still very much in its infancy, yet has massive potential: as the late physicist Richard Feynman once said, ‘what I cannot create I do not understand’.
Already significant headway has been made. In 2010 Daniel Gibson of the Craig Venter Institute chemically synthesized a slightly altered version of the parasitic bacterium, Mycoplasma mycoides, a one million base pair genome, and successfully implanted it into a DNA-free Mycoplasma capricolum cell. Other significant research has been conducted by Prof. Jef Boeke and his collaborators, who are working on a ‘yeast evolution on hyper-speed’3 by creating the first man-made synthetic yeast genome, with the new strain being significantly different from the wild-type strain. This new species will have a range of potential uses from environmental to medical applications.
The first man-made synthetic yeast genome [...] will have a range of potential uses from environmental to medical applications
A Quarter Million Pounder Anyone?
Where could biological engineering go next? The most astounding cell biology project to hit the headlines recently is the production of in vitro meat (IVM). Scientists from the Netherlands led by Prof. Mark Post used stem cell technology to create 140 grams of laboratory cultured beef meat, which was cooked, tasted and critiqued. While it might not yet taste as delicious as a T-bone steak, it is an amazing feat for researchers.
Whilst this work has great implications for the future, the technologies are not yet fully established, and the cost of this research was close to a quarter of a million pounds. Furthermore, the majority of the public made aware of IVM were repelled at the thought of laboratory-grown meat. Hence, while there is potential to turn some vegetarians into omnivores and there are significant potential environmental benefits, in vitro meat is a long way off from reaching the supermarket shelf.
Ethical Concerns
Synthetic biology is still plagued by a number of socio-ethical issues and concerns. Amongst the most relevant are: 1) What if a genetically modified organism was to escape into the environment in an unplanned manner? 2) Could the engineering of living cells fall into the wrong hands – of so-called Bioterrorists? The answer to both these questions is much more complicated than a simple yes or no.
Social scientists look into issues related to the applications of synthetic biology research, and provide us with insights into the dangers, risks and hazards that are associated with ‘new life’. There is now active ongoing research into how to manage GMOs (genetically modified organisms), as well as SOP (standard operating procedures), laboratory health and safety and risk assessments. Plans of action for a variety of scenarios, as well as consideration of what if’s and how to deal with worst case scenarios, have also been developed.
Apart from dedicated social scientists, there are a number of preventative measures to stop non-qualified and non-organizational groups from harnessing synthetic biology in the same way that qualified laboratories achieve this. The US government has created a watch group for these purposes, and supervises the sources that order DNA. Most DNA synthesis companies, such as Life Technology or IDT, will only outsource to registered practicing laboratories. Further discussion of the ethical implications surrounding synthetic biology are publicly available online at the Synthetic Biology Community webpage1.
The fields of synthetic biology and genome engineering continue to produce excellent research, and the potential applications continue to expand as our knowledge and understanding of these respective fields grows. The future holds exciting prospects, and although we are far away from bringing back the mammoth or the dodo, we are one step closer uncovering the fundamental building blocks of human life.